Assessing Cross Contamination Using Layers Of Protection Analysis For Facility And Product Safety
By Patrick Mains, PMP, Theo Zacharatos, and Amanda McFarland, MSc, ValSource, Inc.
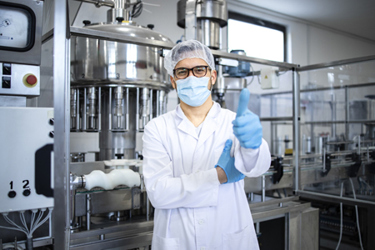
Since ICHQ9(R1) was first adopted, a collection of risk assessment methods has been relied upon to enable hazard identification, risk analysis, risk evaluation, risk control, and review. While the toolkit provided by ICHQ9(R1)1 has a selection of options that range in formality, the guidance document provides a limited selection of risk tools that can aid the industry in complex analyses of controls. When performing the analyses of controls and determining how well those controls are preventing the hazards of concern, considerations that are critical for assessing cross contamination, layers of protection analysis (LOPA) is well suited for the task. LOPA is a risk tool that focuses on the strength of controls that are currently in place and enables a cross-functional team to dive into the ways that the controls may fail. This approach to risk assessment has been used by engineers in the nuclear energy, oil, gas, and chemical industries to determine the facility risks and to determine the safety integrity level (SIL) of safety instrumented systems.2 The same principles that are applied using LOPA in these industries are well-suited for assessing product and facility cross contamination in pharmaceuticals and biopharmaceuticals. A foundational element of LOPA that separates it from other risk tools commonly used is the inherent focus on prevention. LOPA is an ideal tool to proactively assess vulnerabilities against product and facility cross contamination. It focuses on severity and likelihood of product and facility contamination, while providing assurance that each connected area within a facility has controls in place.
In this article, we outline the application of LOPA to product and facility cross contamination.
A Complex, Systematic Analysis Of A Process’s Life Cycle
A function of the LOPA for cross contamination is to determine the potential harms that may result in product or facility cross contamination. Following a raw material through a process life cycle provides a window into the complexity of analysis required. An example raw material flow is provided in Figure 1.
Figure 1: Example Raw Material Flow
Using the LOPA method, the cleanliness controls, equipment, personnel flow, and, ultimately, aseptic technique are assessed to determine the risk to the final product and the facility. Considering the example above, layers of protection are needed from delivery of the raw material to the warehouse through the facility to the production area. Each of the mechanisms of control must be assessed individually to ensure thorough analysis and minimize the opportunity for failure. This includes but is not limited to:
- cleanliness and cleaning practices of materials upon receipt
- preventing material mix-up upon storage in the warehouse
- material and personnel flow paths
- raw material sample handling
- waste flows and waste disposal
To see the forest for the trees, the control analyses using LOPA are performed systematically with a cross-functional team. Each part of the process must be assessed individually and collectively to demonstrate the control strategy is acceptable.
LOPA Strategy
Hazard Identification
The team members selected for a LOPA are critical to gain perspectives on product and facility harm from different areas of operation. Members from the following areas are recommended to ensure completeness of the LOPA: manufacturing, supply chain, microbiology, quality control, quality assurance, validation, shipping/receiving, engineering, and environmental health & safety. Using standard brainstorming techniques, the team of subject matter experts (SMEs) identifies possible hazards (potential sources of harm) that could lead to product or facility harm. Leveraging the ISPE Baseline Pharmaceutical Engineering Guide3, the team works within the framework of the following possible hazard types:
- Mix-up – Materials intended for a certain process or product are inadvertently used for the wrong product.
- Mechanical Transfer – Movement of a cross contaminant on personnel, supplies, or equipment. The cross contaminant must breach the primary container.
- Airborne Transfer – Movement of a cross contaminant through the air/through the HVAC system. This has the potential to move throughout the entire facility. The cross contaminant must breach the primary container.
- Retention – Materials are inadequately removed from a product-contacting surface. Non-product-contacting surfaces also can be considered with the expectation that severities for the different surfaces may not be equivalent.
- Proliferation – This applies to biological products only where the living organisms have a potential for growth. The conditions for growth must be met to realize this hazard.
The next step is to identify the potential causes of each hazard permutation. While not exhaustive, possible causes for hazards may include incorrect or inappropriate cleaning, personnel transfer of the cross contamination, contaminated raw materials or supplies, and poor maintenance or handling of equipment.
Product And Facility Harm
Harm from cross contamination can be viewed as harm to the product and/or facility. The impact of product cross contamination is considered from a manufacturing process perspective where the delivery of drug product to patient is the end goal. Using this strategy, the degree of harm is measured as a function of impact to the patient where the worst-case scenario is a product with cross contamination being released to market. Lesser degrees of patient harm, depending upon the availability of the drug product, may be contamination of drug substance or discarded drug product.
When examining the facility’s risk of cross contamination, harm is evaluated by assessing the level of the breach from the primary containment. For example, can the material in question escape from primary containment and be contained by the secondary containment, or does it escape into the tertiary or quaternary containment zone, or, in the worst possible case, does it contaminate the entire facility? When considering the harm to the facility, it is the ability to contain the breach that is foundational to a preventive approach to cross contamination.
Controls Analysis
Analysis of the controls in LOPA is the foundation of the approach. The controls are, quite specifically, the layers of protection. Performance and success of the LOPA are dependent on the ability of the SMEs to fully assess the current controls that are meant to prevent the cause of the hazard. Example controls, listed in order of effectiveness, include:
- Engineering (E) – These are structural controls that prevent cross contamination, such as segregated operation suites and dedicated HVAC systems.
- Design (D) – This refers to controls regarding the form and fitness of the containment systems, such as a process vessel or final vial. Systems should be designed to enclose the product to protect against loss of containment. Surfaces and equipment should be designed to be cleanable.
- Automation (A) – These are programmable states that prevent cross contamination. Examples are valve sequencing and room interlocks to prevent improper flow patterns.
- Procedural (P) – These are the methods and procedures that are followed each time a process or operation is performed. Examples are wipe downs of materials entering the classified spaces and flow of materials, personnel, product, and waste.
- Training (T) – This refers to formal training on systems, policies, and procedures. Depending on the frequency of performance and monitoring of performance, training by itself should be considered the least reliable type of control. Gowning qualification is an example.
After assembling the information, the content can be displayed in a matrix of the process areas with the hazard types. This will assist the team in understanding gaps in the layers of protection that the facility/product may inherently possess. Table 1 is provided as an example LOPA matrix.
Table 1: Example LOPA Matrix
Determining Cross Contamination Risk
Overall risk is determined by considering the likelihood and severity of harm. Severity is “a measure of the possible consequences of a hazard.”1 When applied to cross contamination, the scaling of severity is a function of the impact of cross contamination, with the most devasting potential impacts being a release of contaminated product or full-scale facility contamination. With a schematic of a facility, the severity can be scaled across the areas where cross contamination can occur. Figure 2 outlines the severity of facility cross contamination as it relates to James Reason’s Swiss Cheese Model.4 Figure 2 illustrates the increase of vulnerability as the material progressively escapes the defined areas of containment, whereas product impact is considered from the perspective of batch-to-batch processing and product-to-product cross contamination.
Figure 2: Facility Layers of Protection
Likelihood is a function of the strength of the controls in place to prevent cross contamination from occurring. To assess the likelihood, the team will consider the controls in place and the effectiveness of the controls as demonstrated by historical data. Using the matrix approach outlined in Table 1, the team determines where controls are lacking and if the existing controls are performing as expected.
A risk parameter that is absent in the analysis of cross contamination is detection. Detection, as a function of the risk equation, is commonly used in failure modes and effects analysis (FMEA) and aids in determining when a failure mode has occurred. The challenge herein lies in the fact that cross contamination is relativity undetectable until final product release and (almost) completely undetectable when it occurs in a facility. For this reason, a focus on preventing cross contamination hazards from occurring and identifying the appropriate strategy to use when those hazards are realized is powerful. For example, if a cross contamination hazard stems from a spill or leak in the facility, identifying a means to prevent the spill/leak from occurring is a key element of strengthening the prevention program. Preventing the contamination from being tracked from one suite of the facility to another is a component of the response strategy.
Conclusion
The LOPA strategy for assessing cross contamination risks must be approached with prevention in mind. Having a robust cross contamination program requires layers of protection to prevent harm to both the facility and product. Using techniques such as process map analysis, control matrix, and the Swiss Cheese Model allow teams to consider the complexity of controls and the effectiveness of the combination of controls. LOPA risk assessments are not static assessments; LOPA requires routine update and review to capture facility changes, control strategy changes, and to incorporate new facility data.
References
- ICH Quality Guideline Q9(R1): Quality Risk Management; International Conference on Harmonization: 2023. www.ich.org
- Handbook of Natural Gas Transmission and Processing, Mokhatab, Poe and Mak. Available online 26 October 2018, Version of Record 26 October 2018, https://www.sciencedirect.com/science/article/abs/pii/B9780128158173000174
- ISPE Baseline Guide: Risk-based Manufacture of Pharmaceutical Products, A Guide to Management Risks Associated with Cross-Contamination, NEED VERSION AND DATE
- Understanding the “Swiss Cheese Model” and Its Application to Patient Safety, Douglass, Wood, Chen and Shappell, Journal of Patient Safety, March 2022
About The Authors:
Patrick Mains is a senior consultant at ValSource Inc. He supports organizations in the pharmaceutical industry using his expertise in quality risk management for pharmaceutical and biopharmaceutical products as well as cell and gene therapies. He holds a BS in biochemistry and cell biology from the University of California, San Diego. Mains is a Project Management Institute (PMI) certified Project Management Professional (PMP). With more than 30 years of experience in the biopharma industry, he has had various roles in quality control, project management, site compliance/inspection management, quality systems, and global quality across multiple organizations. He can be reached at pmains@valsource.com.
Theo Zacharatos is a ValSource Inc. consultant with more than 20 years’ experience in a variety of quality and engineering roles. He has extensive experience within quality, spanning quality risk management, aseptic processing, fill/finish, deviation/CAPA management, process trending, and final product release. He has a BS in chemistry and BE in chemical engineering from New York University and Stevens Institute of Technology, respectively. He can be reached at tzcharatos@valsource.com.
Amanda McFarland is a quality risk management and microbiology senior consultant with ValSource Inc. She specializes in the creation and implementation of risk management programs and developing risk-based strategies for use in clinical and commercial settings. McFarland is an active member of the Parenteral Drug Association (PDA), co-chair of the PDA ANSI standard on QRM in aseptic processing, a member of the PDA Regulatory Affairs and Quality Advisory Board, and an instructor for the PDA. She has a BS in entomology and an MSc in mycology, both from the University of Florida. She can be contacted at amcfarland@valsource.com.