Best Practices For Preformulation In Drug Development
By Andrei Blasko, on behalf of AAPS
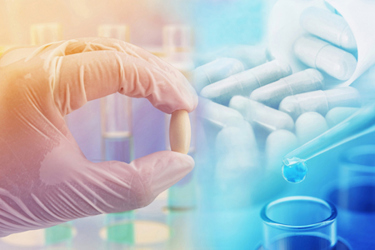
Preformulation testing encompasses all studies performed on a new drug compound in order to produce useful information for subsequent formulation of a stable and biopharmaceutically suitable drug dosage form.1 Preformulation is the connection between drug discovery and drug delivery. Due to the scientific rivalry between drug discovery and drug development, the preformulation group plays the role of peace negotiator. In some companies, the line between preformulation and formulation is a gray zone; keeping them separate leads to great rewards.
There are various stages for preformulation studies during development: preformulation for candidate drug selection at medicinal chemistry (MedChem) or drug discovery stage, preformulation in early development, and preformulation in late development (mainly for extensive characterization, like all possible polymorphs).
The route of administration dictates what is important in preformulation. Ideally, for an oral solid dosage form, a water-soluble, non-hygroscopic, stable polymorph, and easily processed crystalline compound is preferred for development. For solid inhalation compounds, the API needs to be micronized or spray-dried. Micronization is an energy-intensive process that can change the crystallinity and may generate amorphous content and, thus, subsequent interaction with moisture may be important for stability. For a solution formulation, including parenteral and nebulized inhalation products, the stability of the compound in solution will be paramount.
Why Do Preformulation Studies For Drug Selection?
It is useful to conduct preformulation studies and biopharmaceutics studies at the candidate drug selection stage to determine the most relevant physicochemical and biopharmaceutical properties of potential candidate drugs to aid candidate selection. The objective is to achieve a seamless transition from research to development, as opposed to the traditional “over-the-wall” approach that many pharmaceutical companies experience, which adds to their costs. The candidate selection begins in medicinal chemistry’s lead identification (polymorph only), lead optimization and pre-nomination (top three leads), followed by decision on the API, salt, and polymorph (for solids), often referred as pharmaceutical profiling. It is important to have a look at the structural liabilities of the drug candidate as they can predict the long-term stability of the API in the drug product. A physical organic chemist can predict if the candidate will have stability issues and thus will be less likely to make it the market. The candidate selection process needs data on acidity constant(s) (pKa), partition and distribution coefficients (logP, logD), solubility, solution stability, and solid-state characterization: crystallinity, hygroscopicity, solid-state stability, salt selection, and early polymorph screening.
Preformulation In Early Development
From the MedChem stage there is already available data on the API: 1H/13C/2D-NMR, hi-resolution/hi-accuracy mass spec, elemental analysis, HPLC, IR/UV-vis, some solubility/stability information, and hygroscopicity that needs to be shared with the development group.
1. Solubility
Apparent solubility and equilibrium solubility. The physical state of the solute in equilibrium with the solution is a key factor in determining its solubility. Because the solute may cease to exist in its original physical state once it is equilibrated, the physical state cannot have any effect on its equilibrium solubility. Crystal modifications can produce an increase in dissolution rate and a temporary or apparent increase in solubility (kinetic solubility). The effect of crystal structure on solubility lasts only if the modified solid is in equilibrium with the solution. Given sufficient time it will revert to the most stable crystal form (thermodynamic solubility).
Apparent solubility and particle size. The relationship between the radius of a spherical particle and its solubility is given by the Kelvin equation. The Kelvin equation predicts that particles grow with time. Ball milling produces an increase in apparent solubility. Spray drying and freeze drying can be used to produce small particles and can increase apparent solubility.
pH-solubility profile of weak acids and bases (Figure 1). Solubilized weak acids (AH) are mirror images of weak bases (B) and are the sum of the ionized and unionized species. The expression of the dissociation constant Ka leads to the Henderson-Hasselbalch and the solubility equations.
which give the solubility equation for a weak acid,
and a weak base,
where S0 is the intrinsic solubility (solubility of the unionized species).
You can determine the solubility of the salts from pHmax, without first creating the salts.2
Figure 1: pH solubility profile of a weak base
Common ion effect. If an ion in common with the weak electrolyte is added to the solution, the equilibrium is altered (momentarily). To reestablish the equilibrium, some of the salt in solution will precipitate. Adding a common ion reduces the solubility of a slightly soluble electrolyte (important for stability of solid suspensions); the exception is when the common ion forms a complex with the salt. Salts having no ion in common with the slightly soluble electrolyte produce an effect opposite to that of a common ion: at moderate concentration, they increase rather than decrease the solubility because they lower the activity coefficient, but not dramatically.
2. Reaction Kinetics
Reaction (chemical) kinetics is the study of rates of chemical processes and includes investigations of how different experimental conditions can influence the speed of a chemical reaction and yield information about the way the compound degrades. There are a few questions that need to be addressed. For example, how is reaction kinetics helpful in drug development; is this too academic? If you go too deep, it might become academic; however, you need to get the answers you need for your goal, i.e., stabilization of your drug product. To stabilize a drug product, we need to know how the API degrades and what is needed for a reaction to occur: collision (requires mobility; solution vs. solid-state), orientation/proximity, and energy of activation (e.g., heat). To get the answers, pharmaceutical scientists perform forced degradation. The first use of the forced degradation studies is for analytical method development. There is a saying that discovery starts with measurements. The better the measurements, the more reliable the data and the decision process are. Therefore, do not cut corners on forced degradation.
Another question is why do we need to know the pH-rate profile and why do we even need extreme pHs like pH 1 and pH 13? One of the major degradation pathways for a pharmaceutical compound is hydrolysis, and you will find different packaging barriers for humidity protection for the marketed drugs. Therefore, we need to know the pH of maximum stability. But why lower and higher pH values? The answer is that your data helps the analytical development and can give feedback to process chemists, where extreme pHs are used either in sample preparation or in unit operations. In addition, a pH-rate profile that covers the whole pH range can better define the maximum stability (i.e., minimum degradation) and there are multiple cases where the profile is more complex (e.g., bell-shaped curve, Figure 2).
Figure 2: pH-rate profile of adrenaline derivative CpQ5
3. Reaction Mechanism
The sequence of the individual elementary reactions is known as the reaction mechanism. An elementary reaction involves a few molecules, usually one or two. But why do we need to know the reaction mechanism? Understanding how the API degrades helps design the formulation, helps in the identification of degradation products, and helps in analytical method development for structural alerts, where you need to go to ppm level quantitation. Often, a compound’s degradation does not stop at the first reaction step, as in the case of oxidation. However, the first reaction step is important to a drug product’s stability. The reaction mechanism can help you determine this step; kinetics gives you an answer about timing.
Preformulation For Biologics Is Different From That For Small Molecules
Biologics need to be manufactured, shipped, stored, and delivered to the patient, while minimizing their degradation. One of the most concerning degradation pathways is aggregation, leading to immunogenicity that may be triggered with the potential to cause adverse effects in patients. Analytical methods must be developed and validated. Once there is confidence in the analytical data, you can start the formulation development. It is also important to understand how various excipients succeed or fail to stabilize the API during manufacturing and in storage.
The stress conditions usually are milder for biologics vs. small molecules. The first use of the forced degradation of the API is for the stability indicating analytical method development, a must-have tool for the assessment of different formulations.
There are three common modes of denaturation and degradation: aggregation, oxidation, and deamidation. Depending on the complexity of the biomolecule, other types of degradation can take place: isomerization, fragmentation, disulfide scrambling, N- and O-glycosylation, clipping (C-terminal lysine truncation), and pyroglutamate formation. Note: Sample preparation may lead to degradation.
The pH-rate profile determines the pH of maximum stability. Proteins are more stable at their isoelectric point (pI), but the pKa of amino acids and therefore pI, shift with ionic strength; a formulation loaded with ionic species should be avoided and buffer concentration should be minimized.
Forced degradation. In forced degradation we are looking for the major degradation pathways: aggregation, oxidation, deamidation, and photolysis. Aggregation is one of the most scrutinized degradation types for biologics because aggregates may induce an immunogenic response with safety consequences.
Drug–Excipient Compatibility
The performance of the final dosage form (bioavailability and stability) or manufacturability are dependent on the excipients, their concentration, and their interaction with the API and each other. No longer can excipients be regarded simply as inert or inactive ingredients, as described in ICH Q8(R2) Pharmaceutical Development. It is important to know their properties, safety and handling requirements, and regulatory aspects. A list of commonly used excipients in protein formulation has been reported.3 Just because some of these excipients were approved by FDA, it does not mean that they can be used indiscriminately (e.g., glucose is a reducing sugar). Also, most surprises during drug product stability come from excipients, particularly from the impurities in excipients.
Buffers/Salts. The first information you need to know from API degradation is the pH of maximum stability, which leads to the selection of the formulation buffer. The water has no ability to resist change of pH; CO2 from air equilibrated with water changes the pH from 7 to 5.7. It is important to know that some buffers can create problems. Phosphates are Ca‑dependent and Ca salts precipitate. Citrates are chelators, while Tris is a reactive buffer. Buffers like Histidine or Bis-Tris need to be assessed at the pH of maximum stability within ±1 pH unit in 0.5 pH increments. Choose the lowest possible concentration (aggregates form at high µ).
It has been established in the last couple of years that the buffer does not only maintain the appropriate pH of the solution at which the protein is stable in its native structure, it can also influence the stability of the protein itself.4 Buffers recognized as protein stabilizers were Tris, acetate, HEPES, and cacodylate; however, Tris is a reactive buffer and should be avoided, unless there is sufficient data to justify its use.
Sugars. Sugars are exceptionally good stabilizers of proteins, and they are considered as cosolvents. The best choice in protein formulation are non-reducing sugars like sucrose and trehalose. However, trehalose crystallizes during lyophilization; sucrose remains amorphous, but trehalose has a higher glass transition temperature (Tg ≈ 80 °C). The exceptional stabilization effect of trehalose on thermal stability of proteins is attributed to the surface tension effect.
Surfactants. Almost all proteins are adsorbed to a certain extent to surfaces (glass, plastic, metal) and both manufacturing and laboratory analysis had to deal with this property. In addition, the protein solutions at the water/air interface lead to aggregation. Nonionic surfactants are commonly used to avoid extra contribution to the ionic species (e.g., buffers) that may induce aggregation or precipitation.
Antioxidants. Antioxidants help protect against oxidation by scavenging oxygen for themselves. Ascorbic acid is commonly used, but citric acid is preferred, as it is also a pH adjuster. However, some citrates (Fe3+) cause discoloration. Consider for an antioxidant a sacrificing molecule like methionine. The choice of antioxidant is dictated by the liability to oxidation of the API.
References
- Akers, M. J. Can. J. Pharm. Sci. 1976, 11, 1-10
- Mole, J., Box, K., Comer, J. AAPS 2010, https://assets-global.website-files.com/644d2ffb3f7ec1eca95b44dd/64f1891ca1681bab1fe5344c_Sirius-AAPS2010-Measuring-the-solubility-of-salts.pdf
- Capelle, M. A. H. et all. Eur. J. Parm. Biopharm. 2007, 65, 131
- Brudar, S.; Hribar-Lee, B. Biomolecules 2019, 9, 65
- Blasko, A. et all, Biochemistry 2018, 57 (30), 4536-4546, DOI:10.1021/acs.biochem.8b00462
This article is based on the author’s AAPS PharmSci 360 prologue recording.
About The Author:
Andrei Blasko is a subject matter expert in discovering and developing new drugs (small molecules and biologics: mAbs/Fabs, proteins) with 25 years of experience in the pharmaceutical industry. After completing two postdoctoral positions at University of California Santa Barbara, Blasko worked at Roche, Sugen/Pharmacia/Pfizer, Celera, Pain Therapeutics (Cassava Sciences Inc.), and Novartis.
He is a Fellow of the Royal Society of Chemistry (FRSC), editor-in-chief at Journal of Applied Pharmacy, member of the editorial board at American Journal of Advanced Drug Delivery, and he was a FAST advisor at California Life Sciences Institute. After eight years at Novartis, Blasko worked as an independent CMC consultant and in 2021 founded Clearview Pharma Solutions, LLC. He holds seven granted patents.