Critical Considerations In Outsourcing Nanotechnology Drugs
By Barbara Berglund, COO, CMC Turnkey Solutions
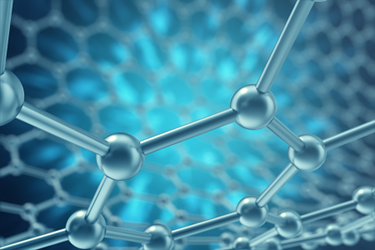
The applications of nanotechnology in medicine and disease treatment are far-reaching and exciting. Targeting diseases and organs, providing vehicles for unstable medicines, and providing innovative therapies are a few examples of these applications. If nanotechnology had a mission statement, it would be “to perform precise interventions at the cellular or molecular level to improve health and promote quality of life.”
INNOVATIONS IN DISEASE TREATMENT
Take, for example, a patient with cancer. With traditional treatment, the therapy used to destroy the cancer cells also harms noncancerous cells. The drug, which is injected or taken orally, passes through the system and organs, is metabolized, and interacts with the entire body. The metabolism factor may mean administering an increased dosage so the drug is effective at the site of interest. Nanotechnology can be used to create a drug that is attracted to the cancer cells and focuses the attack on a tumor instead of the healthy cells a patient desperately needs to rebound and recover. Enzon Pharmaceuticals and Tolmar Pharmaceuticals are among the leading organizations discovering polymeric combinations of drugs to treat cancer. Enzon innovated Oncaspar for leukemia, approved in 1994. With this formulation, the cutting-edge idea of conjugating the protein with a polymer to prolong its activity became one of the first approved nanoproducts for cancer treatment. Tolmar led the treatment of prostate cancer using nanotechnology with its drug Eligard, which was approved in 2002. This drug is a time-release subcutaneous injection. Companies such as Merrimack Pharmaceuticals continue to innovate with drugs such as the pancreatic treatment Onivyde, a liposomal encapsulation that results in delivery of the drug directly to the pancreas, reducing toxicity to other organs. Research at Houston Methodist Research Institute uses traditional cancer drugs encapsulated in silicon microparticles to deliver the drug to the cells, where it is taken up by the target and is efficiently effective (J Control Release).
Another example could be a patient with a blood clot. Traditionally, blood thinners would be given, which impact the entire cardiovascular system. The patient must constantly worry about minor injuries in day-to-day life, as any injury may result in excessive bleeding, causing a critical or even fatal outcome. With nanotechnology, a drug can be crafted that is released only when exposed to shear force, such as passing through a blood clot. With that, the drug is released (and therefore present) only at the site of the clot. At McMaster University in Ontario, Canada, there is ongoing work to create drug surfaces engineered to reduce blood clots. In Italy, a team collaborating across multiple organizations is developing an engineered approach to lysing blood clots.
"Encouraging innovation is an easy sell for biopharmaceutical researchers, but it is more difficult to create the vision to scale up manufacturing."
Consider an unstable drug with a short mechanism of action. The therapeutic application might require hours, days, or weeks, but the drug may be effective only for a few minutes. Traditionally, the therapy would require continuous infusion. Continuous and intermittent infusion therapy is risky, with potential for infection, damaged sites of injection, and over- or underdosing. An infusion pump has complex settings; if they are incorrect, with inaccurate units of dosage over time (e.g., g/hr, units/min, or mEq/kg/hr), human error can cause over- or underdosing. In addition, there can be issues with patient comfort. With nanotechnology, time-release dosing is possible, so the therapeutic agent is present in the correct dosage with single administrations instead of continuous infusion.
For the last decade, cutting-edge research has been used to design DNA origami structures, programmed molecules with precise sizes and structures. Proteins can be fabricated from such DNA structures, removing the need for complicated and multistep syntheses. One such application is the development of improved vaccinations at Karolinska Institutet in Sweden. A vaccine can be developed using this approach by considering what is effective and efficient for the immune system, and then fabricating the precise molecule for ideal effectiveness. This level of research is essential for the future of innovative medicine.
OBSTACLES TO DEVELOPING TECHNOLOGICALLY ENGINEERED MEDICINE
With all the obvious advantages of this kind of targeted therapy, it may seem surprising, at first glance, that there is not more technologically engineered medicine available for patient care. There should be a pipeline of hundreds of novel drugs coming from startups, biotech organizations, and small and Big Pharma to meet the needs in such an effective way. However, multiple hurdles must be overcome to entice a pharmaceutical company to offer nanotechnology alternatives to traditional therapy.
Starting with the financial hurdle, specialized resources are needed from development through scaleup and on to GMP manufacturing. That means there is a need for personnel who are competent to overcome the technical difficulties with such special processing but also with the equipment, which is unique to the process. For the API, processing, and the final drug product, handling considerations and protection of the product and process require esoteric experience.
Take, for example, a process to microencapsulate a cancer drug inside a polymeric microsphere. This process may require the formation of the polymeric microspheres using organic solvents. At the outset, this would require a specialized equipment setup and typically a dedicated space to control handling and organic solvents, such as blast-proof rooms. The equipment may include tangential flow filtration or diafiltration as well. The removal of organic solvents is critical for the ultimate safety of the product.
"Multiple hurdles must be overcome to entice a pharmaceutical company to offer nanotechnology alternatives to traditional therapy."
An organization seeking to outsource such a process needs a partner with either facilities in place or a willingness to collaborate and create the appropriate design to support the program with long-term goals in mind. This means build-outs, specialized designs, blast-proofing, and support utilities to handle organic solvents and evaporation of these solvents. This also means laboratory support and possibly outsourcing some specialized testing. The expense of this kind of partnership is considerable on both sides and represents organizational risk. For a new clinical product or a pipeline of products, it may be a decade before a profit is realized on either side. Further, a contract manufacturer that performs a build-out for a process may not be able to use the specialized space for any other process, depending upon the level of specialization implemented during build-out.
Now consider, for this microencapsulation process, the regulatory and quality risks. The regulatory strategy needs to include a defendable position on how the new therapy is an improvement on traditional treatments. Exciting words like “cutting-edge technology” are met with a conservative review from regulatory bodies, which are concerned with patient safety and mitigating risk at all times. The quality summary needs to embrace product as well as process controls; for a microencapsulated product, maintaining sterility while handling solvents is a concern. Some organic solvents cannot be sterile-filtered. Also, with microspheres, understanding the critical quality attributes such as particle size requires a solid understanding of the quality boundaries of the drug product. Controls around par ticle size need to be implemented and well-explained in the regulatory package. Development and validation of product-specific test procedures are essential, and a specialized field. The process-validation parameters for each step of the process need to be explained, and a strong program for controls needs to be included in the regulatory package.
Encouraging innovation is an easy sell for biopharmaceutical researchers, but it is more difficult to create the vision to scale up manufacturing. The key to overall success is to set up compliant manufacturing facilities that can be agile with nanotechnology. Long-term planning, keeping the ultimate payoff in mind, is needed.
Perhaps a CMO begins by repurposing a manufacturing area for one focus on nanotechnology. Perhaps this is, as in the example, setting up utilities and blast-proofing for handling organic solvents, then hiring the right workforce or training the workforce in place to gain the knowledge and understanding of this kind of processing. The workforce includes manufacturing, maintenance, laboratory, quality, and regulatory personnel. It requires a well-designed calibration and maintenance program for unique equipment. Having some basic equipment in place but having, as the business model, an openness to adding appropriate monitoring and processing equipment is needed. This openness requires agility and adaptability, as each nanotechnology process is unique.
The outcome for all is a platform for providing novel medicines and therapies. The bottom-line financial gain may trail behind the design by years, but providing services in this space is a universal need. If the mission statement of nanotechnology is “to perform precise interventions at the cellular or molecular level to improve health and promote quality of life,” then the contract manufacturer might seek a mission statement such as, “to enable novel therapy in a safe, effective way, advancing medical science one medicine at a time.”
BARBARA BERGLUND is COO of CMC Turnkey Solutions, where she applies over 20 years of experience in finished pharmaceutical, API, and medical device manufacturing.