Impact Of The Freeze-Drying Process And Lyoprotectants On Nanoparticle Stability
By Dr. Xiuling Lu Ph.D.
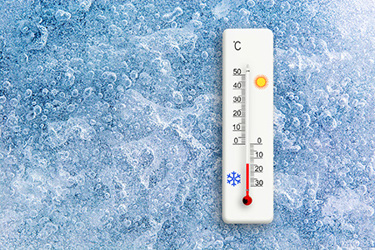
Nanoparticles are extremely diverse, ranging from solid lipid structures to liposomes and even designs that incorporate gold/ silica particles. One of the recent roles of nanoparticles is for the delivery of drugs, for cancer treatments. Their complex structures are usually core-shell based where a drug is encapsulated by the nanoparticle and transported throughout the body. These can be directed to a specific area of the body, reducing toxicity and increasing the effectiveness of the drug. A recent example is the use of lipid nanoparticles (LNPs) to wrap SARS-CoV-2 mRNA as a basis of several vaccines recently developed for COVID.
Most nanoparticle formulations are in solution with a heterogeneous size distribution. These nanosuspensions can be unstable and subject to problems, such as sedimentation, agglomeration, crystal growth or chemical reactions. Lyophilization can increase the stability of these particles, which also benefits the stability of the drug products incorporated in them.
Recently, Dr. Xiuling Lu, University of Connecticut, presented a webinar describing the challenges of freeze-drying nanoparticles and how, in collaboration with Dr. Robin Bogner Ph.D., her group has designed and evaluated solutions to lyophilizing LNPs. This tech note summarizes the webinar and includes a selection of questions from the Q&A session.
Challenges of Lyophilization
There are many challenges in the freeze-drying process, some of which are due to ice formation during freezing which can cause particle aggregation or structural damage to the nanoparticles. The resulting cake morphology is also sensitive to specific glass transition and collapse temperatures (Tg’ and Tc, respectively) during cycle development.
Even after the freeze-drying process has been optimized, the reconstitution time needs to be considered and made as short as possible for convenient administration of the drug product by the end-user.
Lyophilization conditions for each nanoparticle and drug can be improved by evaluating and optimizing these parameters and using lyoprotectants. Ideally, an optimal lyophilized product would exhibit elegant cake morphology with no collapse, maintain the structure of nanoparticles and drug entrapment, contain low moisture (<2%) for long term stability, and be reconstituted in a short time.
Dr. Lu examined the freeze-drying conditions for three different nanoparticles: solid lipid nanoparticles (SLNs), polymeric nanoparticles (PNs), and liposomes (Lipos). The Tg’ and Tc for the three nanoparticles were determined with a range of lyoprotectants, before investigating the impact of formulation and freezing conditions on the quality of the nanoparticles, and the long-term stability of the final product.
A LyoStar 3 freeze dryer with ControLyo® Technology (SP) was used for these experiments which enabled ice nucleation to be controlled and all vials to be frozen simultaneously.
I: Impact of Formulation
Three lyoprotectants were compared for their effect on preserving the freeze-dried nanoparticles (SLNs, PNs and Lipos).
- Sucrose (1:5 and 1:10)
- Trehalose (1:5 and 1:10)
- Mannitol (1:5 and 1:10)
The addition of any of the lyoprotectants did not result in significant changes in cake morphology, with reasonable cakes produced without collapse in any vials. However, there was evidence of shrinkage in some vials, in particular liposomes without lyoprotectants.
Although the elegance of the freeze-dried cakes was similar, the reconstitution time was greatly reduced by the addition of a lyoprotectant. In PNs, mannitol (1:5 and 1:10) reduced this time from 35 minutes to less than 5 min (Figure 1).
Successful lyophilization of the nanoparticles was also evaluated by measuring particle size and homogeneity (polydispersity index, PDI) before and after freeze-drying. The lyoprotectants preserved the particle size of SLN and Lipos with sucrose and trehalose being more effective than mannitol. In PNs, the particles’ size was not greatly affected by freeze-drying, with or without lyoprotectants. It is possible that the polyvinyl alcohol (PVA) present in the PN formulation may provide some protection from aggregation.
Sucrose and trehalose were the most effective lyoprotectants at maintaining particle sizes and improving size distribution. SLNs and Lipos need lyoprotectants to maintain size distribution, but PNs need lyoprotectants to shorten the reconstitution time.
Figure 1: Impact of the addition of a lyoprotectant on reconstitution time
II: Impact of Freezing Conditions
Freezing is a critical step in the lyophilization process. Many problems can occur due to uncontrolled freezing, such as heterogeneity in ice crystal size within the batch, and a higher risk of collapse caused by minute ice crystals
ControLyo® is a technology that controls nucleation, enabling a higher nucleation temperature, which generates larger crystals leading to faster primary drying times.
In this study, the effect of different freezing conditions was compared for each of the nanoparticles
- Uncontrolled ice nucleation with a cooling rate at 1C/min to -40C
- Flash-freezing - particles are immersed into liquid nitrogen before placing in freeze-dryer for drying
- Slow freezing - controlled ice nucleation using ControLyo® at -4 °C and cooling rate of 0.2 °C/ min to -40 °C
- Controlled ice nucleation using ControLyo® at -4 °C and -8 °C cooling rate at 1 °C/ min to -40 °C
Under flash-freezing conditions, all the products exhibited extremely serious cracks and all the Lipos without lyoprotectant collapsed. Uncontrolled ice nucleation also generated significant cake shrinkage with the Lipos.
Slow freezing was the only freezing condition that increased both the particle size and homogeneity (PDI) of the SLNs and Lipos with lyoprotectants (Figure 2). However, it did not affect the size or size distribution of PNs, possibly due to the presence of PVA, as described previously.
Figure 2: Impact of freezing conditions: results-solid lipid nanoparticles
The freezing conditions also did not affect the reconstitution time and residual moisture content for all nanoparticles, although addition of an effective lyoprotectant reduced both parameters for all nanoparticles.
Ice nucleation temperature is generally lower in aseptic cleanrooms due to the controlled environment to meet ISO 5 or Grade A classification.
III: Long Term Stability
Different storage conditions were compared to determine long term stability of nanoparticles.
- Liquid formulation at room temperature (RT)
- Liquid formulation at 4 °C
- Freeze-dried with uncontrolled ice nucleation at RT
- Freeze-dried with controlled ice nucleation at RT
Storing SLNs in liquid form at RT dramatically enlarged the particle size by 100% in 10 days (Figure 3) and rapidly increased the PDI. Storing the SLNs in a fridge at 4 °C was slightly better and a 100% increase in particle size was observed at three months. The storage of this nanoparticle was significantly improved by freeze-drying, and when using a controlled ice nucleation method, there was no change in particle size at three months. Data was not present for Lipos at this current time.
Figure 3: Change in Mean Particle Size of SLNs under Different Freeze-Drying and Storage Conditions
In contrast, the size and homogeneity of PNs were not significantly affected by any of the storage conditions as they were relatively stable in all conditions
Conclusion
Freeze-drying nanoparticles with controlled ice nucleation at -4 or -8 °C provides better cake morphology without collapse, lyoprotectants enable preservation of particle size, improved particle homogeneity, and shorter reconstitution time. However, these conditions differ between different nanoparticles, so it is important to optimize each product separately and not assume conditions are interchangeable.
As the predominate reason for freeze-drying a product is long term stability, careful evaluation of lyophilization conditions for each nanoparticle will ultimately benefit the manufacturing and storage capacity for many drug and vaccine products, such as the COVID-19 mRNA Vaccine BNT162b2 from Pfizer that currently requires storage at -80 °C making it challenging to distribute and administer appropriately.
To view the full webinar and download the slides, please go to the archived webinars on our website ... https://www.spscientific.com/Webinars/Archives/.
Q&A Session
1. For liposomes, since it has water inside the liposome, is the optimal moisture residual still lower than 2%, or should be higher?
To the best of our knowledge, there are no specific residual moisture recommendations established for freeze-dried liposomes. Even if there is water inside liposomes, we did not see a higher residual moisture in freeze-dried liposomes than other nanoparticles from our results, implying that the intraliposomal water may be insignificant with respect to the overall residual moisture of the freeze-dried product.
2. What is the concentration of NP studied and reported in your presentation?
The concentration of SLNs, PNs, and liposomes are 5 mg/mL, 5 mg/mL, and 12.5 mg/mL respectively.
3. What methods were used to analyze the residual water of freeze-dried particle?
Karl Fischer titration was used to determine the residual moisture, and anhydrous methanol was added to the freeze-dried nanoparticles to disrupt their structures.
4. Are the liposomes, PNs and SLNs used in the studies pegylated?
No, none of them is PEGylated.
5. Could you please describe or repeat the reconstitution method?
The samples were reconstituted using 1 mL of water. A syringe and a needle were used to inject the water through the center of the stopper into the vial. The vial was manually swirled at 90 rpm on the circumference of a circle with a diameter of 11 cm. Swirling was paused intermittently after certain time intervals for 15 seconds of visual observation. The swirling was stopped until no unsuspended cake solids in the vial was found. Total reconstitution time included the observational pause periods, because the cake was in contact with water during that time.
6. How much PVA was in the PLGA NP?
1.5% PVA solution was used in PLGA NP formulation.
7. Did you try to optimize the cryoprotectant ratio to any of the nanoparticles?
We compared a few ratios and found that 1:10 (NPs:lyoprotectant) showed satisfactory lyoprotective properties in SLNs and Lipos. A ratio of 1:5 is minimally required.
8. Beside size/PDI, any impact on % encapsulation by lyo process and by cryoprotectants?
The impact of lyophilization process or lyoprotectants on drug encapsulation was not reported in this study, but it is currently being studied by our group.
9. Thanks for the great presentation. Which phospholipid did you use for lipos?
L-α-hydrogenated soy phosphatidylcholine.
10.Can you share the details of the lyophilization cycle that was used for all of these samples?
Samples were first cooled at 1°C/min to 5°C and held for 30 minutes, followed by cooling to −8°C. Ice nucleation was induced at this point by ControLyo technique. The shelf was then cooled to −40°C at 1°C/min and held for 30 minutes to complete freezing. For primary drying, the chamber pressure was set at 50 mTorr, and the shelf temperature was elevated to −20°C at 0.2°C/min, and held until the reading of the Pirani gauge converged to that of the capacitance manometer. For secondary drying, the shelf was then heated to 30°C at 0.2°C/min, and held for 4 hours.