Model-based Control In Continuous Manufacturing Of Biotherapeutics: The Role Of Process Integration
By Anurag S. Rathore, Saxena Nikita, and Garima Thakur
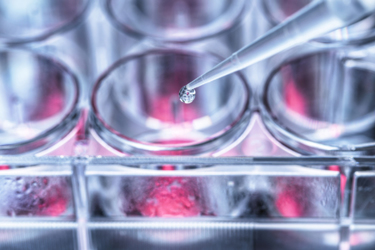
In our previous article, we discussed the prerequisites for model-based control and its implementation to enable real-time control of various unit operations. These model-based techniques can be used for creating an end-to-end integrated continuous manufacturing platform. In comparison to batch manufacturing, continuous manufacturing offers shorter development time, improved and uniform product quality, lower capital cost, and easy scale-ups. Hence, the main focus is to satisfy critical quality attribute specifications. Four key elements of continuous process are continuous flow, end-to-end integration, system approach, and an integrated control system. Furthermore, it is evident from the published literature that integrating upstream and downstream process platforms is more complex than just connecting all the unit operations in series. Modifications in flow rate, loading volumes, process conditions, and handling process deviations are required.1,2 The primary focus, thereby, lies on establishing a flexible and standard platform with real-time monitoring, automated start-up/shut down, and a control strategy to address the dynamically changing conditions.
Upstream and downstream should be integrated in such a way that bioreactor output is continuously processed in downstream. A suggested approach is to integrate the bioreactor with clarification/cell retention devices followed by continuous capture chromatography. Here, cell retention devices can be alternating tangential flow filtration (ATF)3,4 or tangential flow filtration (TFF). Mechanistic models of these devices help in comparing their performance and thereby in designing the optimal filtration cascade.3,5,6 Apart from filtration devices, centrifuge is another option that can employed for clarification. Studies on disc stack centrifuge applying a computational fluid dynamics (CFD) model to relate cell lysis with stresses indicated that productivity increases with reduction in cell lysis and cell damage is more if the rotational speed is high.7 In another study, a model was used to predict the rate of change of bed height in fluidized bed centrifuges.8 Owing to the recent advancements, acoustophoretic separation can also be used as retention devices.9 For continuous operation, cadence acoustic wave separators (Pall Life Sciences, NY) can be used for enhanced cell separation.10 Such modelling studies demonstrate the effect of critical process parameters (CPP) on cell separation efficiency. They are also useful for gaining deeper understanding of the unit operation and in developing fully automated large-scale continuous cell clarification systems. An integrated platform comprising a capture process (two-column continuous process), viral inactivation process, polishing step (semi continuous, twin column), followed by batch wise flow through the polishing step, has been developed and demonstrated.11 Customized design principles based on mass balance, along with deterministic and statistical models to develop control strategies, have been designed to directly connect the unit operations. Another architecture of end-to-end continuous bioprocessing involves a perfusion bioreactor in combination with ATF, one four-column periodic counter-current chromatography PCC for capture and viral inactivation, followed by another PCC for intermediate and polish steps.12
While integrating adjacent downstream unit operations, the discontinuous nature of the downstream process also needs to be considered. It is important to have a provision to absorb fluctuations in flow rate from the upstream units and provide a constant flow to the downstream process. Surge tanks are one solution to the inherent periodic nature of unit operations, making realization of directly connected integrated processes possible.13 The flow scheme of an integrated bioprocess is shown in Figure 1, wherein surge tanks, also known as hold tanks, are used to create an end-to-end platform. They help to maintain low operating pressure14 and perform real-time control of process deviations arising due to titer change, charge variant change, or membrane fouling conditions.15-18 Many platforms for integrating unit operations include operating the chromatography process in periodic fashion,19 with hold tanks in between to provide constant flow to the downstream operations, or placing a surge tank between the perfusion bioreactor and adding a continuous capture step to control the flow rate fluctuations from upstream to downstream unit operations.20 Here, at-line HPLC, coupled with the mechanistic model, is also used to control and optimize the capture process. In another study, an integrated platform comprising a perfusion bioreactor combined with ATF, capture multicolumn chromatography, viral inactivation, single pass TFF, polishing membrane, viral filtration, and single pass UF DF, along with three surge tanks, resulted in 110 g/l final concentration, in comparison to 85 g/l in batch manufacturing.21 These surge tanks assisted in pressure relief. However, surge tank use is not encouraged because capital cost, hold time, productivity, and operation are all negatively affected.11 Most of the studies mentioned here have been validated for small-scale biological therapeutics production (minimum 3.5 days to maximum 31 days).
Figure 1: Example of an end-to-end continuous platform created by integrating multiple unit operations using surge tanks
Current Status of Integrated Continuous Bioprocessing
Recent advancements in bioprocess manufacturing are augmenting process development and productivity; however, new challenges arise when implementing them. Solutions are required in the operational, control (for both process development control challenges and process control challenges), and validation aspects to improve productivity and efficiency. Bioreactor operation requires efficient consideration of process development, process control, and characterization time, taking into account the process’ operational complexity and contamination risks. These concerns can be seen as high and significant for perfusion operation. Single-use perfusion units are only recently available, and lack of understanding presents additional challenges. Companies like Cytiva, Sartorius, and MilliporeSigma have developed automated bioreactors and new perfusion systems. Transcenta’s integrated bioprocessing platform uses continuous perfusion to obtain high productivity in addition to eliminating clarification and the hold step. This technique, in combination with robust process control strategy and automation, reduced footprint, labor, consumables, cycle time, etc. The process output was increased by tenfold.22
In downstream unit operations, adoption of continuous chromatography is challenging because an integrated control structure is required for its operation. However, the availability of equipment with built-in data systems is enabling continuous operations. For products difficult to produce using traditional methodology, upstream perfusion in combination with multi-column chromatography (MCC), in-line conditioning, and TFF are being used. Recently, advancements in affinity methods have boosted productivity; for example, Nanopareil’s electrospun nanofibers, membrane capsules, and monoliths offer short residence time and high dynamic binding capacity. It improved Protein A chromatography as well as polishing chromatography by offering fast cycle times, reduced chromatography facility footprint, and reduced buffer consumption and waste generation. On a broader scale, a fundamental problem in adoption of upstream and downstream continuous manufacturing is the inability to integrate the unit operations. Although many options for creating an integrated platform are available (as discussed in the previous section), the applicability of these platforms at a larger scale needs to be assessed.
Conclusion
Further research is needed on the topics of process control, real-time monitoring, automation, knowledge management, flexible infrastructure to reduce carbon footprint and capital investment, simplified technology transfer, and improving supply partnership management. Developing a comprehensive model or digital twin for the entire chain will help in designing an optimized process and making real-time decisions to automatize the process. Real-life examples of the same are yet awaited. Further, opportunities lie in establishing a flexible approach with automated systems and mathematical models to enable real-time process monitoring and control. Finally, regulatory approaches that can adapt to the needs of continuous manufacturing need to further evolve. Regulatory guidance is needed for single-use standards, process validation strategies, data integrity, interface protocols, and vendor specifications.
References
- Konstantinov, Konstantin B, and Charles L Cooney. “White paper on continuous bioprocessing. May 20-21, 2014 Continuous Manufacturing Symposium.” Journal of pharmaceutical sciences vol. 104,3 (2015): 813-20. doi:10.1002/jps.24268
- Rathore, Anurag S, et al. “Challenges in Process Control for Continuous Processing for Production of Monoclonal Antibody Products.” Current Opinion in Chemical Engineering, vol. 31, 2021, p. 100671., https://doi.org/10.1016/j.coche.2021.100671.
- Karst, D.J. et al. “Characterization and comparison of ATF and TFF in stirred bioreactors for continuous mammalian cell culture processes” Biochem. Eng. J., 2013, 110:17–26.
- Warikoo, V. et al. “Integrated continuous production of recombinant therapeutic proteins” Biotechnol. Bioeng. 2012, 109, 3018–3029
- Hadpe, S. R. et al. “ATF for cell culture harvest clarification: mechanistic modelling and comparison with TFF” Journal of Chemical Technology & Biotechnology, 92(4), 2017, 732-740. doi: https://doi.org/10.1002/jctb.5165
- Walther J, et al. “The effects of alternating tangential flow (ATF) residence time, hydrodynamic stress, and filtration flux on high‐density perfusion cell culture” Biotechnol. Bioeng. 116, 2019, 320–332.
- Shekhawat, Lalitha K, et al. “Application of CFD in Bioprocessing: Separation of mammalian cells using disc stack centrifuge during production of biotherapeutics” J. Biotechnol. 267, 2018, 1–11.
- Kelly W, et al. “Understanding and modeling retention of mammalian cells in fluidized bed centrifuges” Biotechnol. Prog. 32, 2016, 1520–1530.
- Shirgaonkar, I. Z., et al. “Acoustic cell filter: a proven cell retention technology for perfusion of animal cell cultures” Biotechnol. Adv. 22, 2004, 433–444.
- Banerjee, S, et al. “Mechanistic modelling of Chinese hamster ovary cell clarification using acoustic wave separator” Chemical Engineering Science, Volume 246, 2021, 116894, https://doi.org/10.1016/j.ces.2021.116894.
- Steinebach, F., et al. “Design and operation of a continuous integrated monoclonal antibody production process”. Biotechnology progress 33, 5 (2017), 1303-1313.
- Godawat, Rahul et al. “End-to-end integrated fully continuous production of recombinant monoclonal antibodies.” Journal of biotechnology vol. 213 (2015): 13-9. doi:10.1016/j.jbiotec.2015.06.393
- Thakur, Garima et al. “Control of surge tanks for continuous manufacturing of monoclonal antibodies.” Biotechnology and bioengineering vol. 118,5 (2021): 1913-1931. doi:10.1002/bit.27706
- Hernandez, R, To Surge or Not to Surge? Genetic Engineering & Biotechnology News vol. 17, 16 (2015), 120-130.
- Rathore Anurag S, “Roadmap for implementation of quality by design (QbD) for biotechnology products”. Trends Biotechnol. 27 (2009) 546–553.
- Read, E. K, et al. “Process analytical technology (PAT) for biopharmaceutical products: Part I. concepts and applications”. Biotechnology and Bioengineering, 105, 2 (2010), 276–284
- Rathore, Anurag S, and Winkle H, “Quality by design for biopharmaceuticals,” Nat Biotechnol, vol. 27, no. 1 (2009), pp. 26–34
- Rathore, Anurag S, et al. “Process analytical technology (PAT) for biopharmaceutical products”. Analytical and Bioanalytical Chemistry, 398, 1 (2010) 137–154.
- Gomis-Fons, Joaquín et al. “Model-based design and control of a small-scale integrated continuous end-to-end mAb platform.” Biotechnology progress vol. 36,4 (2020): e2995. doi:10.1002/btpr.2995
- Karst, Daniel J et al. “Process performance and product quality in an integrated continuous antibody production process.” Biotechnology and bioengineering vol. 114,2 (2017): 298-307. doi:10.1002/bit.26069
- Arnold, Lindsay et al. “Implementation of Fully Integrated Continuous Antibody Processing: Effects on Productivity and COGm.” Biotechnology journal vol. 14,2 (2019): e1800061. doi:10.1002/biot.201800061
- Shanley, A., “Moving Closer to End-to-End Continuous Bioprocessing,” BioPharm International 34 (5) 2021.
About The Authors:
Anurag S. Rathore, Ph.D., is an Institute Chair Professor at the Department of Chemical Engineering, Indian Institute of Technology, Delhi, India. He is also the coordinator for the Centre of Excellence for Biopharmaceutical Technology and is presently serving as IIT Delhi’s Dean of corporate relations. His previous roles included management positions at Amgen Inc. and Pharmacia Corp. His areas of interest include process development, scale-up, technology transfer, process validation, biosimilars, continuous processing, process analytical technology, and quality by design. He has authored more than 500 publications and presentations in these areas. He is presently serving as the Editor-in-Chief of Preparative Biochemistry and Biotechnology and Associate Editor for Journal of Chemical Technology and Biotechnology. He has a Ph.D. in chemical engineering from Yale University.
Saxena Nikita is research associate working under Prof. Anurag Rathore at Indian Institute of Technology, Delhi. She is an expert in process control and is presently working on automation and digitization of biopharma processes for continuous production of biopharmaceuticals. Her areas of interest include process integration, model-based control, statistical process control, and implementation of multivariate data analytical techniques for process monitoring and control. She has published more than 20 articles and a book related to control of various processes, and has filed two patents in the biopharmaceutical field.
Garima Thakur is a researcher working under Prof. Anurag Rathore in the Bioprocessing Lab at the Indian Institute of Technology, Delhi. She works in the area of PAT applications for continuous downstream manufacturing of biopharmaceuticals and has authored more than 10 publications and four patent filings in the field. Her work is focused on overcoming the unique challenges associated with continuous operation in the biopharma industry, particularly handling process deviations with real-time control tools. She has worked on developing novel PAT tools, including data-driven AI/ML solutions and novel spectroscopic tools for improved control of unit operations in the continuous downstream manufacturing train.