Rapid Microbiological Methods & Alternatives To Colony Forming Units: A Pathway To Implementation?
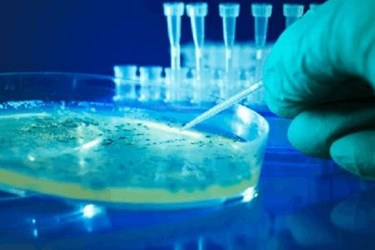
By Hans-Joachim Anders (Novartis), Fred Ayers (Eli Lilly), Carol Davis (Shire), Brian Fitch (Biogen), Deborah Gessell-Lee (Baxter), Scott Hooper (Merck & Co.), Michelle Luebke (Baxter), Jeanne Mateffy (Amgen), Lisa Yan (Shire), and Jeffrey Weber (Pfizer Inc.) of the Online Water Bioburden Analyzer Workgroup
The challenges of comparing conventional colony forming units (CFUs) to newer technologies that are capable of counting individual cells or measuring different variables/factors (such as light, shape, or fluorescence) has limited the acceptance and implementation of new technologies in the pharmaceutical industry. The CFU is the historical “gold standard” of microbiology but is recognized to have many limitations with respect to microorganism recovery rates and time to results. Additionally, individual users may employ challenge microorganisms for rapid microbiological method assessment that are unique to a facility or process; this results in varying comparisons due to microbiological differences based on handling, passages, and preparation.
We propose the path forward is to have internationally recognized non-microbiological standards to serve as benchmarks that can be used to compare system performance to representative microorganism recovery. This paper will describe the technique and quality oversight required for successful implementation of rapid microbiological methods based on measures other than the CFU.
Background On Rapid Microbiological Methods (RMMs)
Rapid microbiological methods (RMMs) have been widely available for more than two decades, but there has been limited uptake into monitoring routine pharmaceutical manufacturing. Attempts to rationalize the slow acceptance and implementation of RMMs have led to speculation that has had a further dampening effect on the uptake of these promising technologies. The primary roles envisioned for RMMs have been in high-value and high-risk applications, such as pooling steps or monitoring hold times.
Automation of current growth-based compendial methods and method advances for detection and enumeration of microbial colonies tend to utilize the CFU as the conventional reporting units. There have been documented applications where exhaustive comparisons were performed using solid phase cytometry, but those are detailed and expensive comparison studies.1 Several industry guidance documents have been published (PDA Technical Report 33, USP <1223>, and EP 5.1.6), but these may lack sufficient information to comprehensively validate a novel method; nor are regulators required to accept these guidance recommendations.
Limited work has been done to provide industry-wide harmonization of CFUs to the alternative measures used by the various RMMs. We propose the implementation of a certified reference material (CRM) surrogate fluorescent particle standard with one (or more) characterized bead(s) as a means for normalization across the field. This concept could also be applied to other units of measure (i.e., relative light units [RLU] or cells).
Shortcomings Of Colony Forming Units (CFUs)
In microbiology, a colony-forming unit is an entity used to quantify the magnitude of viable bacteria or fungal populations within an individual sample. Viable is defined as the ability to replicate under controlled conditions. CFU enumeration requires microbial growth and counts only viable cells, in contrast with microscopic examination, which can count all cells, living or dead. The appearance of a colony requires logarithmic growth before is it visible to the naked eye, and though accepted as a base assumption, it remains uncertain whether the colony arose from a single cell.2 Since the colony may have arisen from a group of cells, expressing the result in terms of a CFU propagates this uncertainty.
The growth-based agar plate count estimates the microbial population within a sample utilizing microorganisms' capability to replicate under the applied medium, temperature, and time conditions. One viable cell can give rise to a colony through replication. However, solitary cells are the exception in nature, and frequently the progenitor of a colony is a mass of cells deposited together. In addition, many bacteria grow in chains (e.g., Streptococcus) or clumps (e.g., Staphylococcus) and form biofilms.
Biofilms propagate by shedding cells into a flowing stream; the ability to monitor low-level random microorganisms facilitates early detection of biofilm formation. Estimation of microbial numbers using the CFU underestimates the true number of viable cells present in a sample — the theory that every colony is separate and founded by a single viable microbial cell is not demonstrated in situ.
The large number of variables involved in the preparation of a viable microbial standard is challenging and includes: species/strain selection, number of passages, cellular adhesion, recovery rates, and microbial growth phase. Testing protocols vary from company to company, making comparisons across sites and locations variable and difficult. The preparation of a microbial standard is often a reflection of analyst technique as much as instrument response. We propose that a CRM provides an “anchor point” against which testing can be benchmarked. Figure 1 illustrates the benefits of a reference point for multivariate calibrations.3
Figure 1: The analysis demonstrates the challenges of comparing multi-variable components (size, number, etc.) of different microbes compared to a CRM (Bin B). The CRM provides a fixed reference point for relative response of the instrument and operator variability. Bin A and C represent two different sample populations compared to the CRM standard (Bin B).
Bio-Fluorescent Particle Counts (BFPCs) — An RMM Alternative
Bio-fluorescent particle counts (BFPCs) is a novel technology based on intrinsic biological auto-fluorescence of microorganisms. The approach uses a laser (typically 405 nm) to excite biological molecules (e.g., ATP, NADH, and picolinic acid) and detect the resulting emitted fluorescence. This technique is used for both air and liquid samples. The moving sample stream passes through an optical cell, and the analyzer continuously monitors the detection frequency, particle size, and bio-fluorescence. The opportunity to use BFPC to provide continuous and rapid signals of changes in the manufacturing areas, either air monitoring or water loop monitoring, provides significant preventive and process-diagnostic value.
Based on the challenges encountered in identifying a suitable and reliable standard to validate BFPC instruments, we sought an industry collaboration with the National Institutes of Standards and Technology (NIST) to characterize a fluorescent bead particle that can be used to calibrate and compare instrument response to various microorganisms.4 NIST provides an independent verification of the number, size, and fluorescence of vendor-supplied particles. The standard beads can be used to demonstrate the appropriate system responses during installation, operation, and performance qualification. The use of a CRM provides standardization and therefore confidence in the implementation of RMMs. Additionally, standard beads can be used to verify system operation without the need to remove the instrument from the manufacturing area; the use of a CRM eliminates a key hazard associated with introducing microorganisms in manufacturing areas.
Conclusion
Standardized benchmarks are points of reference from which physical, intellectual, operational, or analytical space can be mapped back to the agreed-upon reference point. The implementation of a standardized CRM will provide vendors, industry, and regulators with a consistent calibration and suitability testing benchmark standard for harmonization of RMM assessments based on BFPC. The use of an inert material for calibration and periodic suitability testing will create confidence needed to improve and accelerate adoption of RMMs in the pharmaceutical industry for users, vendors, and regulatory agencies.
References:
- R. Smith, M. Von Tress, C. Tubb, et al., “Evaluation of the ChemScan RDI as a Rapid Alternative to the Pharmacopoeial Sterility Test Method: Comparison of the Limits of Detection,” PDA Journal of Pharmaceutical Science and Technology, 64 (4), 356–363, 2010.
- S. Sutton, “Counting Colonies,” Pharmacuetical Microbiology Forum Newsletter, Vol 12(9), 2-10, Sep. 2006.
- D. Marie, “Analysis of picoplankton by flow cytometry,” Plancton Océanique Roscoff, Jan. 1998.
- R.A. Hoffman, L. Wang, M. Bigos, J.P. Nolan, “NIST/ISAC Standardization Study: Variability in Assignment of Intensity Values to Fluorescence Standard Beads and in Cross Calibration of Standard Beads to Hard Dyed Beads,” Cytometry Part A, 81A:785-796, Sep. 2012.
About The Authors:
The authors are members of a collaborative workgroup formed to guide the development and implementation of an online water bioburden analyzer (OWBA). By leveraging lessons learned through assessment and implementation of various rapid microbiological methods (RMM) and process analytical technologies (PAT) across the healthcare industry, the primary goal of this workgroup is to provide guidance regarding the development of OWBA systems that would be broadly accepted by the industry and regulators. To facilitate this goal and engage instrument vendors, the OWBA workgroup has developed three key documents — a user requirements specification (URS), a testing protocol, and a business benefits proposal. The URS provides fundamental OWBA system design inputs, as well as additional desirable attributes. The protocol provides a list of verification and performance tests which the vendor can apply to demonstrate system capability and operation. The business benefit guidance provides the financial justification for both vendors and industry to drive the successful implementation of an OWBA platform.