Process Analytical Technology: Benefits Of Spectroscopic Tool Use
By John D. Orr, Ph.D., and George L. Reid, III, Ph.D.
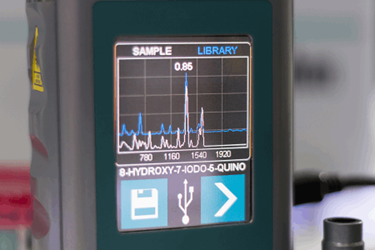
The first column of this three-part series provided an introduction to process analytical technology (PAT) and discussed the challenges associated with its adoption and implementation by industry. One challenge noted was the lack of substantial corporate exposure to PAT.
Pharmaceutical companies have made significant investments in analytical technologies, such as chromatography (high-performance liquid chromatography [HPLC] and gas chromatography [GC], for example), over the years. As a result, there is extensive (multi-decade) individual, organizational, and regulatory agency experience, knowledge, and comfort with these technologies and the resulting data. In contrast, there is much less experience and comfort with the use of spectroscopy and PAT, resulting in an unfamiliarity with how, when, or why to use the tools. Also, including a process control or release method in an NDA filing, based on technologies with limited pharmaceutical precedence, presents a real or perceived risk to product approval timing. This lack of familiarity and perceived risk can result in an organizational or management reluctance to use new technologies on critical projects. These (organizational and individual) human factors are considerable obstacles to overcome.
To introduce new technologies into an organization, the financial and human investment (including hiring or training of staff) associated with the purchase must be justified. This is not simple, as there are often budget constraints and many competing proposals that are more critical or can have a more immediate return on investment. For example, the choice often comes down to investing in new technologies versus replacing obsolete or malfunctioning equipment essential to the continuity of organizational operations (e.g., replacing a reactor needed to produce drug substance). Therefore, discussions on prioritizing capital proposals often boil down to funding a “must have” over funding a “would like to have.”
Prior to PAT introduction, the process chemist or engineer typically independently ran processes, withdrew samples, and delivered them to the analytical chemist for analysis. The analytical chemist would analyze the samples using an offline method, resulting in a time delay between sampling and results. These results would then be used by the process chemist or engineer to evaluate the process conditions tested and determine possible next experiments. This approach can be considered pharmaceutical development via an iterative scientific assembly line. Chemists create, analysts test and provide results, the chemist evaluates the data and creates a new sample for analysis. After several iterations, the process is refined and set. With this arrangement, barriers exist between different groups that are working as a team toward the same goal of developing a well-understood and controlled process.
Once the company decides to make an initial investment in PAT, an instrument is typically brought in as a research tool in a non-GMP setting. Via this approach, a modest research and development investment with minimal overall risk takes place; further, no impact to GMP operations is incurred. PAT couples the process with the analytical tool, thus the analytical chemist, process chemist, and engineer must collaborate directly (to install the technology, utilize the equipment, run the process, and evaluate the data). The scientist and project team collect in situ data and compare it with traditional offline analytical data to determine the suitability of the results and, hence, the potential value of the new technology. The comparison of the online and offline data often results in the PAT instrument providing valuable insights (e.g., transient species, mechanistic information) into the progression of the reaction that are not seen in traditional analyses (where reactions are quenched prior to analysis). Thus, the use of PAT introduces a perturbation to the way process development has traditionally been conducted within pharmaceutical development organizations, and its use represents a positive step toward technological modernization and catching up with process tool usage in other industries (e.g., chemical, petrochemical, and food). A best practice for process development and PAT occurs when the disciplines of analytical chemistry, chemical engineering, and process chemistry come together as a single team with a common goal.
Once PAT is applied and used, additional process knowledge that is highly useful to the project team may be gained in the laboratory setting. While the influx of information can be a bit overwhelming, there are some lower-risk opportunities to gain comfort with spectroscopic tools that are commonly used in PAT. For example, by evaluating and implementing the spectroscopic and PAT tools early during candidate development and in the early manufacturing process steps, the risk of PAT use is mitigated via time and distance from the commercial product.
This article will provide examples and describe the benefits of performing spectroscopic measurements in lieu of more traditional analyses to provide familiarity with the PAT tools, data, and processed results. PAT applications will be discussed in the third article.
Analytical Technology Selection Considerations
The first step in analytical method development is to determine what data is needed. Examples include material identity, completion of a chemical reaction, verification and control of a continuous process, and material drying.
The second step is to consider the process that will be monitored and the associated requirements of the analytical technique (e.g., the speed, selectivity, sensitivity, accuracy, and precision with which the measurement must be made). It follows naturally that a technique will be selected that can measure the desired property, has the required performance, and is available. In many cases, more than one technique (traditional and PAT) can be selected to provide the requisite data.
To provide some familiarity with PAT (spectroscopic) techniques, several illustrative examples of typical pharmaceutical analyses follow.
Identity Testing
Raw materials and excipients are routinely received at manufacturing sites, sampled, and sent to a lab for confirmation of identification (using IR [infrared], NMR [nuclear magnetic resonance], or HPLC techniques). Sampling the materials can lead to worker exposure, as well as the potential for material contamination. Handheld spectrometers containing a library of material spectra can be used to confirm material identity at the loading dock, and often through the optically transparent plastic liner or glass container. Data analysis within the spectrometer compares spectra to produce an identity result.
The use of a handheld spectrometer for identity testing of incoming materials used in pharmaceutical manufacturing (drug substance and drug product) is demonstrated in the following video from Metrohm. In this example, solids were analyzed through a plastic liner, and liquids were analyzed through an amber glass bottle and a clear vial via use of a handheld Raman spectrometer. This allowed for rapid and safe material identity confirmation, while ensuring material integrity. Other suppliers of similar devices include B&W Tek, Thermo Fisher Scientific, Agilent, and Bruker.
Concentration Verification In Static Systems
Samples are typically consumed when conducting traditional analyses. However, as shown above, it is possible to obtain analytical information via non-destructive means. There are numerous examples of non-destructive measurements that can be made spectroscopically, and several sample applications follow.
During the process of drug substance and drug product manufacturing, various solutions require preparation. Errors in solution preparation can result in manufacturing process errors or failures. Therefore, the verification of desired solution composition is of value. Solvent mixture composition, for example, can be confirmed via near infrared (NIR) spectroscopy, as described in a NIR method developed for measuring water content in acetone.
An important step in processing involves dissolving a solid substance to create a solution of a desired concentration. In cases where the material to be dissolved absorbs UV light, a UV/Vis probe (such as an ATR [attenuated total reflectance] UV/Vis probe) can be used to confirm desired solute concentration has been reached, as illustrated in an ATR-UV/Vis method used to measure dissolved caffeine concentrations.
Historically, most medicines were based upon small molecules. With advances in biotechnology in recent decades, numerous important pharmaceutical products are protein-based and are produced as freeze-dried powers to ensure material stability. Therefore, monitoring drug product moisture levels during lyophilization is important. Several different techniques can be used to measure lyophile moisture content. Near-infrared spectroscopy (NIRS), for example, can be used to determine moisture levels in lyophiles (through the glass vial) without the need to open the vial.
Concentration Determination In Dynamic Systems
Monitoring of closed systems (e.g., chemical reactors) is important during process development and manufacturing. Chemical reactions often involve use of highly reactive chemical species and are often performed under temperature and pressure extremes and pose a threat to worker safety during traditional sampling procedures. Chemical reactions are often sensitive to atmospheric oxygen and moisture, thus putting the reactions at risk of perturbance or failure during traditional sampling procedures. Additionally, many chemical reactions occur so rapidly that sampling and analyzing them using traditional techniques is simply too time-consuming and not practical.
Liabilities are associated with sampling chemical reactions via traditional means. These risks can, however, be reduced or eliminated using in situ (within the reactor) reaction-monitoring techniques (e.g., PAT). The ability to “see” within the reactor in real time with or without minimal sampling and offline testing provides a wealth of data without worker exposure or reaction perturbation risks. Using in situ monitoring with infrared absorption spectroscopy, information about reaction initiation, mechanism (via the detection of a transient intermediate), and completion can be gained, as illustrated in this video from Mettler-Toledo. ABB and Bruker offer similar technologies. These PAT tools can also be used to monitor material dissolution, material precipitation or crystallization, and polymorph control.
Conclusions
To increase familiarity with PAT technologies, this article provides examples that illustrate where traditional analytical tests could be supplemented, improved, or replaced with these new tools, such as identity testing, concentration verification, and concentration monitoring (in real time). It also introduced the types of analytical tools that are used in PAT. In these examples, the emphasis was placed on the “AT” in “PAT.” However, in PAT, the analytical technologies (like those introduced here) are interfaced with processes (the “P” in “PAT”), and the analytical data is used in real-time process control.
The final article in this series describes examples where industry has succeeded in meeting the 2004 U.S. FDA aspirational guidance for industry on process analytical technology.
About The Authors:
John D. Orr, Ph.D., managing advisor with Rondaxe Pharma, LLC, has 25 years of pharmaceutical chemistry, manufacturing, and controls (CMC) experience. He has been with Rondaxe since January 2016, assisting clients in the areas of CMC analytical and process development. Prior to joining Rondaxe, John served as director of analytical and physical chemistry at Eisai, where his group specialized in the analytical characterization and pharmaceutical development of complex natural product-based medicines and protein-based therapeutics.
John has been active in several industry groups, serving on the board of directors of the IQ Consortium and the Allotrope Foundation. John received his chemistry degrees from the University of Pittsburgh (B.S.) and The University of Chicago (M.S. and Ph.D.), and performed post-doctoral studies in molecular biology at The Noble Foundation. You can reach him at johnorr0105@gmail.com or connect with him via LinkedIn.
George L. Reid, III, Ph.D., principal consultant with Cardinal Health Regulatory Sciences, has more than 20 years of pharmaceutical CMC experience, including regulatory, candidate progression from preclinical to post-approval, and technology development. He has contributed to several approved medicines and works with clients in the areas analytical, drug substance, and formulation development.
George has been active in industry groups and currently is on the planning committee for the Land O'Lakes Pharmaceutical Analysis Conference. George earned doctorate in chemistry from Missouri University of Science and Technology and a B.S. in biochemistry from Beloit College. You can reach him at george.reid@cardinalhealth.com or connect with him via LinkedIn.